While electricity producers have traditionally focused on transporting electrons and oil & gas companies on molecules, green hydrogen holds the potential to blur these distinctions. As the energy landscape evolves, green hydrogen could significantly disrupt the competitive balance between these two industries. It could herald the emergence of an ‘electricity first’ energy ecosystem, marking a shift from the ‘fuel-first’ system prevalent in past centuries. Should this transformation occur, 21st-century electricity producers could ascend to a level of influence similar to that held by fossil fuel producers in the 20th century.
Historical roles of utilities vs oil & gas producers
In the past century, electric utilities and producers were just a subset of the global energy system. Petroleum-based products, in various forms, were extracted from the earth, processed, and transported to diverse end-use markets. Some of these products were used for propulsion in transportation or as heat sources in industrial processes. Others were sold to utilities to be burned, transformed into electricity, and then distributed to end users.
Our entire energy ecosystem, including electricity, has been historically rooted in petroleum exploration and production. As a result, oil and gas companies have emerged as some of the largest and most influential corporations globally. While utility companies can be substantial, they generally have smaller market capitalizations compared to the supermajors in the oil and gas sector. Among the top 100 companies in the world, from a market capitalization perspective, ten are oil & gas companies, while none are ‘electricity first’ companies. This disparity in size between oil and gas companies and utility companies is primarily due to the differences in the commodities they deal with and the scale of their operations. Utility companies are typically more regionally or nationally focused, serving local or regional markets. In contrast, oil and gas companies operate on a global scale, with extensive operations and investments across various countries and regions.
An evolving energy landscape
Shifting to renewable electricity and an ‘electricity first’ society will be crucial for the long-term sustainability of our energy system. Over the past two decades, wind and solar have emerged as viable technologies, yet their market shares remain relatively small. In 2019, less than 10% of global primary energy was derived from electricity generated by wind, solar, and hydroelectric power (see Figure 2). While there are ambitious projections for a rapid shift in the energy mix in the coming decades, these projections also highlight critical challenges that must be addressed to ensure a resilient, reliable, and affordable global energy supply.
Renewable energy sources like solar, wind, and hydropower are crucial in the global transition to cleaner and more sustainable energy systems. However, they have limitations, and understanding these is essential for a realistic assessment of their role in the energy landscape. The primary limitations of renewable energy include:
- Intermittency and variability: The intermittency and variability of renewable energy sources, such as solar and wind, are perhaps their most significant limitations. These sources are dependent on weather conditions and daylight hours. Solar power is unavailable at night, and wind energy is inconsistent. This creates challenges in maintaining a stable and reliable energy supply, necessitating backup power sources or energy storage solutions to bridge the gaps.
- Energy storage costs: To mitigate the intermittency issue, energy storage technologies like batteries are required. While storage solutions are advancing, they can be expensive to deploy and maintain. This cost can be a significant limitation, particularly for large-scale grid integration of renewables.
- Grid integration challenges: Integrating renewables into existing electrical grids can be technically challenging, often requiring infrastructure upgrades to manage their intermittent and decentralized nature. Furthermore, power distribution over long distances from remote renewable sites to urban areas can result in energy losses and increased costs.
- Limitations on EVs: Range anxiety is a common concern, as electric vehicles (EVs) typically have a more limited driving range compared to traditional gasoline vehicles. Charging infrastructure remains a challenge in some areas, requiring more widespread and faster charging options. Over time, battery degradation can impact EV performance and longevity.
- Industrial electrification, while vital for reducing emissions, has its limitations. High-energy industries, such as steel and cement production, face specific challenges. Electric furnaces in steelmaking, for example, may require substantial energy input and face technological barriers. Moreover, electrification in these sectors often calls for significant infrastructure upgrades and a reliable electricity supply, with grid capacity constraints being a potential limitation.
Solving the limitations of ‘electricity first’
The reliability and availability of fossil fuels, which we must aim to replicate in an ‘electricity-first’ world, can be achieved through green hydrogen, a critical linchpin in this transition. As the main input of green hydrogen is green electricity, it provides a vital path to extend the benefits of electrification, representing a form of ‘indirect electrification’. Direct electrification opportunities, such as EVs, buildings, and low-temperature heat pumps, have clear, positive value propositions and are increasingly adopted worldwide. Whenever possible, direct electrification is the way to go. These methods are more energy-efficient than burning fuels. However, we also require non-fossil fuel solutions for other energy needs like heavy-duty transportation, firm power generation, and industrial use. Green hydrogen can provide this flexibility and drive significant demand for more renewable energy installations.
To transition to a hydrogen-powered economy, achieving low-cost production is not enough. Investment is needed in infrastructure for storing and transporting hydrogen. Building an efficient hydrogen transportation network, akin to the century-long development of fossil fuel infrastructure, will take decades. While this infrastructure is being deployed, Power-to-X (PtX) technologies provide a critical transition solution over the next decade. The conversion of hydrogen into synthetic hydrocarbons, often referred to as e-fuels or electrofuels, could revolutionize our energy system by replacing fossil fuels with an ‘electricity-first’ approach. PtX technologies involve converting renewable electricity and water to hydrogen with electrolysis. The hydrogen is then combined with captured carbon dioxide (CO2) to generate synthetic hydrocarbons such as synthetic gasoline, diesel, or natural gas. These e-fuels can be utilized in existing infrastructure, engines, and industrial processes, offering a seamless transition from fossil fuels.
The role of synthetic hydrocarbons in the energy transition
- Decarbonizing transportation: One of the most significant challenges in the energy transition is reducing emissions in the transportation sector. While electric vehicles (EVs) are on the rise, synthetic hydrocarbons offer a viable decarbonization path for sectors like aviation, shipping, and heavy-duty transportation. E-fuels can replace fossil fuels without requiring extensive changes to existing infrastructure or engine technologies.
- Energy storage and grid balancing: Synthetic hydrocarbons can serve as a form of energy storage. Converting excess renewable electricity into e-fuels during high-generation periods and then back into electricity during peak demand helps address the intermittency of renewable sources. The fuels can stabilize the grid, ensuring a reliable and constant energy supply.
- Carbon neutrality and recycling CO2: Synthetic hydrocarbons can be produced using captured CO2 from industrial processes or direct air capture. This means they can be carbon-neutral when the CO2 emissions released during combustion are balanced by the CO2 captured during production. This closed-loop system contributes to carbon recycling and reduces net greenhouse gas emissions.
- Sustainable chemicals and industrial processes: Synthetic hydrocarbons can replace fossil-based feedstocks in chemical and industrial applications. By using e-fuels, various industries can reduce their carbon footprint and contribute to the sustainability of their products and processes.
Addressing the storage and transportation issues of renewable electricity, as well as the challenges of industrial electrification, can position electric utilities and renewable energy producers as leaders in the global energy ecosystem by 2050. According to the International Renewable Energy Association’s Net Zero projection, renewable electricity could power over 60% of global energy use (see Figure 3). Achieving this goal requires flexible solutions for utilizing clean electricity, with green hydrogen playing a key role in developing this flexibility.
The hurdles to indirect electrification with green hydrogen
Although green hydrogen holds promise for transitioning to a renewable energy economy, economically affordable production technology is not yet available. The key cost factor in hydrogen production via electrolysis is the OPEX of electricity consumption. Electrolysis requires a significant amount of electricity, contributing to nearly 75% of the cost per kilogram of hydrogen in many cases. Despite efforts to enhance electrolyzer efficiency, there is a limit to potential improvements. Notably, many electrolyzers already achieve about 80% efficiency from a Higher Heating Value perspective (hydrogen HHV is 39.4 kWh/kg). Therefore, figuring out how to access lower-cost electricity for electrolysis might be more impactful than focusing on efficiency.
To put the importance of price of electricity in perspective, if you were given a 100% efficient electrolyzer for free, but had to use $70/MWh electricity, the hydrogen produced would cost around $3/kg. Most studies show we need to produce hydrogen for between $1–2/kg to avoid extra costs in our energy system.
The high OPEX of electricity for hydrogen production underscores the need for low-cost electricity sources. In the U.S., one of the best opportunities for this is behind-the-meter integration with renewable energy, where Power Purchase Agreements (PPAs) with new onshore wind installations can range from $20–30/MWh, according to the U.S. DOE’s 2022 Land-Based Wind Market Report. At these rates, hydrogen could be produced at less than $2/kg with an 80% efficient electrolyzer. While efficiency is less critical in this scenario, the CAPEX of the electrolyzer becomes much more important. Renewable energy resources typically achieve 25–40% utilization rates, emphasizing the importance of each dollar spent on CAPEX for project success. Technology R&D should be focused on low-cost, load-following electrolyzers to meet our hydrogen goals. Alkaline electrolyzers need to improve their operational variability, PEM electrolyzers should reduce precious metal usage to lower CAPEX, and AEM electrolyzers must continue demonstrating their commercial durability if they are to deliver a low-CAPEX, load-following option.
The road ahead
Electric vehicles have become one of the most significant drivers of green electrons for electricity producers in the last century. Similarly, green hydrogen presents renewable energy developers with an opportunity to further develop clean energy sources, thereby extending the renewable energy value chain. The road to gaining superiority in the energy landscape with green hydrogen is filled with both opportunities and challenges. As the global energy landscape shifts towards sustainability, electricity producers have a unique role to play in driving in this transformation. By investing in green hydrogen production, infrastructure development, and innovative applications, they can harness the potential of this clean energy carrier to reduce carbon emissions and diversify their revenue streams.
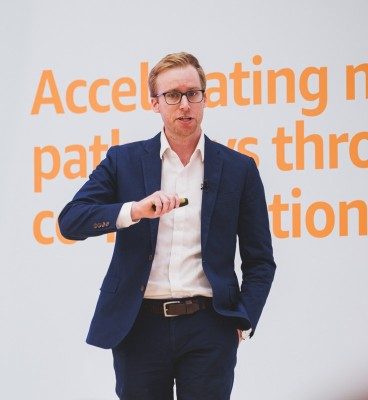