By Amit Khedkar and Dr Ahmed Halima, Mott MacDonald
Electrolyser readiness for economy of scale
Assessing electrolyser readiness through benchmarking the confirmed large-scale project pipeline against completed projects provides a critical measure of the state of electrolyser technologies and progress of their commercial application.
As of the end of January 2023, the hydrogen industry has announced more than 1,000 large-scale projects requiring electrolyser capacities of more than 1 MW. Among these projects, 795 aim to be fully or partially commissioned by 2030.1
Moreover, gigawatt-scale projects are gaining momentum, accounting for 112 developments, which include 91 for hydrogen production from renewable energy and 21 for low-carbon hydrogen production.
This ever-increasing global demand, illustrated in Figure 2, shows that hydrogen production is ramping up towards economies of scale. However, this poses some challenges on the demand of electrolyser technology types to suit different requirements and applications across developments.
Electrolyser types and the race to 2050
There are several types of existing and novel electrolysers with varying technology and commercial readiness levels. Alkaline (ALK) and Polymer Electrolyte Membrane (PEM) electrolysers are commercially available, while technological advancements are facilitating the development of new types of combined-heat electrolysers in the early stages.
In most countries, announced developments with ALK technology currently exceed PEM. In contrast to the USA, where PEM electrolysers account for 45% of installed and announced electrolyser projects, Australia relies more on PEM electrolysers, potentially due to a higher reliance on behind-the-meter renewable electricity sources, anticipated cost reduction, and lower maintenance requirements.
ALK technologies are better suited for stable and power-firmed electricity sources, such as from the grid, compared to PEM, which can be installed with variable power without impacting the electrolyser performance. Figure 3 shows the distribution of electrolyser technologies in emerging hydrogen economies worldwide, based on data from the IEA Hydrogen Projects Database.2
At this stage in the emerging hydrogen economy, announced projects are proposing an increasing number of electrolyser capacities, and developers are looking to establish a pipeline of hydrogen projects to scale up based on demand by implementing project expansion capabilities. This requires electrolysers to adopt modularisation approach for scale-up. Table 1 summarises the hydrogen product capacities for key Original Equipment Manufacturers (OEMs) that can modularise to higher capacities.
Considering the advantages of PEM electrolysers over ALK, which include simpler balance of plant, sizing, and footprint, as well as high-pressure hydrogen production, the cost breakdowns of hydrogen plant engineering and development vary for both technologies, as shown in an example study depicted in Figure 4. While long-term cost reduction of electrolyser technology is anticipated, it is important to consider the overall cost of engineering and balance of plant when planning and assessing the feasibility of a commercial project.
Electrolyser and catalyst materials development
Electrolyser technologies have experienced a significant rise in new developments over the past several years as a result of the emerging hydrogen economy. In 2016, the number of patents related to electrolysis surpassed those for technologies related to liquid hydrocarbon feedstocks, and this trend is expected to continue growing in the coming years.4
New technological advancements in electrolysers are empowering the industry in two ways: through the development of new and innovative technologies such as Solid Oxide Electrolyser Cells (SOEC) and Anion Exchange Membrane (AEM) electrolysis, which are gaining focus.
While the current market is still dominated by ALK technology, in the future, not only are PEM electrolysers expected to play a major role, but SOEC as well, pending commercial demonstration in prolonged operation. Technological advancements will likely contribute to innovation in hydrogen electrolysers, leading to improved efficiencies, better gas and thermal management, and high-load flexibility for commercial electrolysers, among other parameters, as illustrated in Figure 5.
The increase in efficient production and cost reduction of electrolysers supports industry trends focused on improving efficiencies and reducing costs in the future. This includes the cost of hydrogen plants, which is expected to reduce by 40% in the near term and by 80% in the long term. Additionally, there are expected increases in the technological performance and durability of hydrogen electrolysers.
To ensure the reliability and long-term success of electrolysers, the following factors are key:
- Prioritising durability and longevity of hydrogen electrolysers through materials selection, thermal management, and robust product design.
- Considering reliability from the perspectives of design, monitoring, operation, and safety.
- Implementing new solutions to enable real-time collection and analysis of data from electrolysers, allowing for the identification of patterns and trends that may indicate the need for maintenance or repair.
At the core component of an electrolyser is the cell stack which is responsible for the electrochemical process. For the cell to operate, it requires a replenished electrolyte, which can be in solid or liquid form depending on the type of electrolyser.
Traditional ALK electrolysers have additional requirements, including lye separators, storage, and reticulation components. Older ALK technology has already improved to zero gap by including a porous transport layer and catalyst layer in the membrane electrode assembly, leading to enhanced power density and cost reduction. As a result, ALK electrolysers now use a similar stack structure as PEM electrolysers.6
Table 2 presents a summary of the materials used in the manufacturing of stack components for both technologies. Some critical scarce materials used in both technologies, as shown in Figure 6, pose significant supply chain risks. These materials include iridium, platinum, and gold. Therefore, technological development is necessary to identify and test non-noble metal electrocatalysts for hydrogen production.
Policies and regulations to support electrolyser manufacturing
An estimated 35–40% of the global hydrogen demand is expected to be met by water electrolysis by 2040. As of September 2022, 25 countries, along with the European Commission, have released hydrogen strategies.7 Leading governments in hydrogen policy include the United States, United Kingdom, Japan, Chile, France, Germany, and the European Union.7
Policies that directly support electrolyser manufacturers typically focus on R&D and process improvements. To make the hydrogen economy more feasible, additional policies are needed to promote the sale of green hydrogen, including subsidies with a ‘Green Label’ designation.
Government and regulatory bodies also play a key role in facilitating the establishment of codes and certification standards that are used to define the legal framework for hydrogen technologies. In the Asia-Pacific region, several regulatory initiatives are underway, including the development of standards such as ME-093 by Standards Australia.8 Additionally, certification of origin, such as the Guarantee of Origin by the Australian Clean Energy Regulator,9 is being implemented both nationally and globally by international and European standards organisations.
Currently, the market is pursuing large-scale projects in the megawatt range, and OEMs are meeting this demand by developing scalable electrolyser stacks ranging from 1 MW to 5 MW. Based on lessons learnt through hydrogen feasibility and front-end engineering projects, as well as engagement with project developers and OEMs, the following summarises some of the challenges that the industry faces in green hydrogen plant developments.
Availability of components and raw materials
ALK components require no scarce or noble metals; however, PEM components require Platinum Group Metals (PGM), including platinum and iridium, as shown in Figure 6. Recent import and export sanctions, resulting from ongoing global political unrest, have led to congested supply chains and limited availability of raw materials and components.
Similarly, material shortages caused by congested supply chains are also affecting the computer chip industry, which, in turn, impacts the manufacturing of electronic equipment and balance of plant.
Capacity for scaling up and electrolyser availability
Although commercially available, installed and proven at small scale, ALK and PEM technologies need to demonstrate viability and deployment to integrate with multiple end-use applications and infrastructure at scale and commercially viable costs.
Longevity and durability for high performance at scale
At megawatt scale, electrolysers require durability testing and demonstration at this scale for prolonged operation to validate their lifespan for various applications. There are potentially unknown factors, such as variable ramp-up of load issues and power stability, that need to be addressed.
Energy density and sizing
Critical challenges for ALK include the power density of stacks, enlarging the partial load range, reducing system size and complexity/footprint, and improving the dynamics of the overall system. ALK electrolysers exhibit a lower power density per footprint ratio compared to PEM, making the overall system footprint more space consuming, which is a challenge considering the already constrained availability of land10 and associated costs.
High-pressure operation
High-pressure production directly from the electrolyser will be a key driver for cost reduction for ALK electrolysers, as PEM electrolysers already provide advanced solutions for high-pressure hydrogen production compared to ALK.
Hydrogen purification
Currently, Pressure Swing Adsorption (PSA) and Temperature Swing Adsorption (TSA) technologies are used for hydrogen purification. Further improvements in hydrogen purification technologies, including innovative technology development such as Vacuum Pressure Swing Adsorption with compression, are needed.
Availability of water
This is a challenge for all electrolyser technologies. Developers need to assess alternative sources of water at a large scale and consider pathways for wastewater treatment or desalination, which incurs an added cost for water processing and treatment. Currently, approximately 9 litres of water is required per kilogram of hydrogen production without considering losses from the water treatment unit and cooling water requirements.
Shared infrastructure assets and government policy
Besides market demand, challenges in renewable hydrogen production at a large scale also arise from the need for enabling infrastructure, shared assets, and supportive government policies. These factors significantly impact the progress of the hydrogen economy and pose challenges for proponents. Policies and standards are not yet finalised in many countries, or in many cases, they are not user-friendly for a smoother transition.
Cost
PEM electrolysers are currently more expensive (10–20% higher than ALK) due to the cost of noble metal catalysts. &D development is required to identify cheaper alternative catalytic materials. PEM and ALK cost parity is anticipated by 2030, considering the current scale-up of development and high demand.
Notes & references
1 Hydrogen Council & McKinsey & Company. (May 2023). Hydrogen Insights.
2 The International Energy Agency (IEA) produced the Hydrogen Project Database to track advances in low-carbon hydrogen technology. The dataset covers all projects commissioned worldwide since 2000 to produce hydrogen for energy or climate-change-mitigation purposes. Projects in planning or construction are also covered.
7 IEA. (2022). Global Hydrogen Review 2022.
8 Standards Australia. ME-093: Hydrogen technologies.
About Mott MacDonald
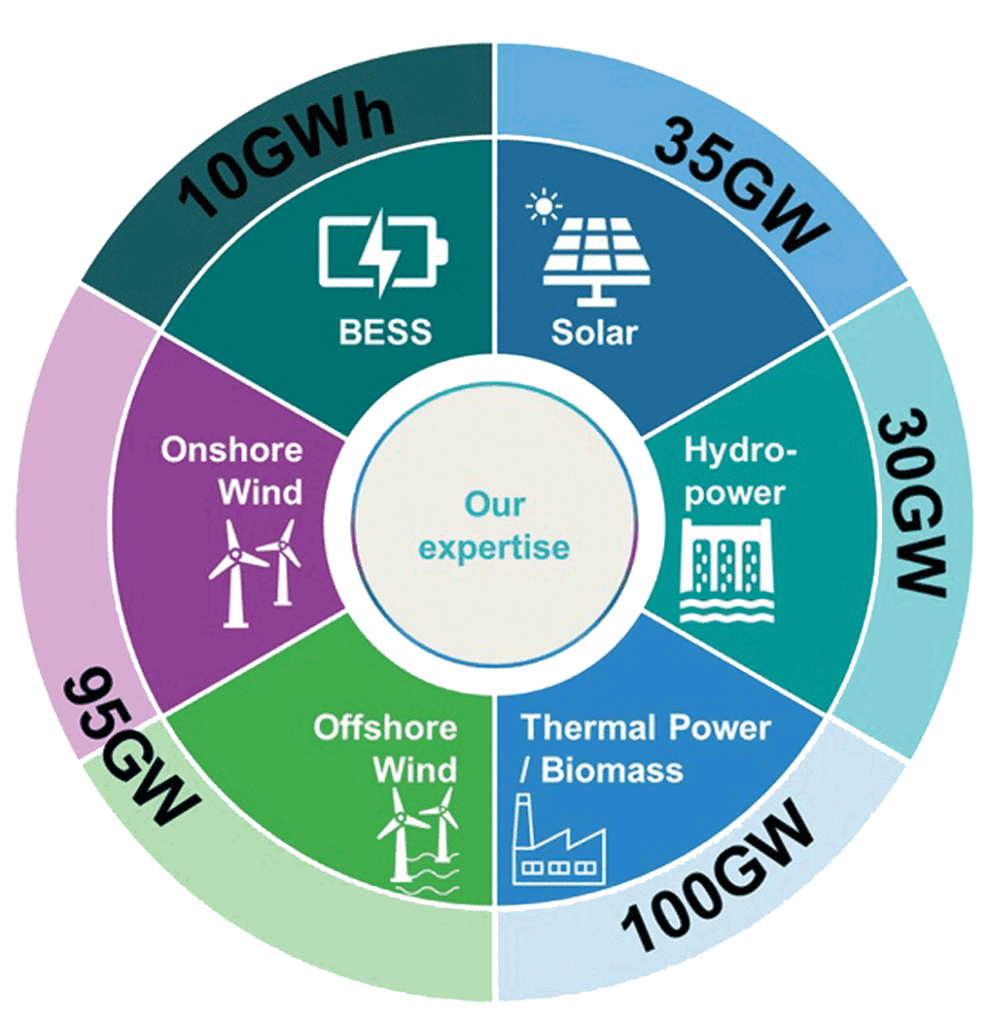
Mott MacDonald is a global engineering and advisory management consulting firm specialising in energy, transport, infrastructure, water, and the environment. Our focus on guiding clients and delivering solutions to some of the planet’s most intricate challenges promotes positive social outcomes and supports sustainable targets. Our track record in the Asia-Pacific region, including Australia and New Zealand encompasses feasibility studies and front-end engineering solutions for various applications in energy, transport, industry, and cross-sector projects. To find out more, visit www.mottmac.com.